Biomedical imaging plays a vital role in modern medicine and biological research. From diagnosing diseases to monitoring treatment responses and conducting basic research, the quality of biomedical images directly affects the accuracy and reliability of research results.
The optical filters used in imaging systems are an important component that significantly affects imaging quality. Filters help improve image quality by selectively transmitting or blocking specific wavelengths of light, reducing noise, and highlighting desired features of target tissues.
Types of Filters in Biomedical Imaging
There are various types of optical filters used in biomedical imaging, each designed for a specific purpose based on its spectral properties. The most commonly used filters include broadband filters, narrowband filters, bandpass filters, and longpass and shortpass filters.
1. Broadband Filters
Broadband filters transmit a wide range of wavelengths, making them ideal for applications that require capturing a broad spectrum of light, such as white light illumination in microscopy. These filters are versatile and can cover most of the spectrum, from ultraviolet (UV) to infrared (IR).
However, the main disadvantage of broadband filters is that they tend to introduce more background noise, which can obscure the details of the target features.
2. Narrowband Filters
Narrowband filters are used to transmit a very narrow range of wavelengths, usually centered around a specific peak wavelength. They are particularly useful in fluorescence microscopy and other imaging techniques where the goal is to isolate a single emission peak from a fluorescent dye or marker.
Narrowband filters have high specificity, allowing precise selection of the desired wavelength while blocking out-of-band light. However, their narrow transmission windows can reduce signal strength, which can be a limitation in low-light conditions.
3. Bandpass Filters
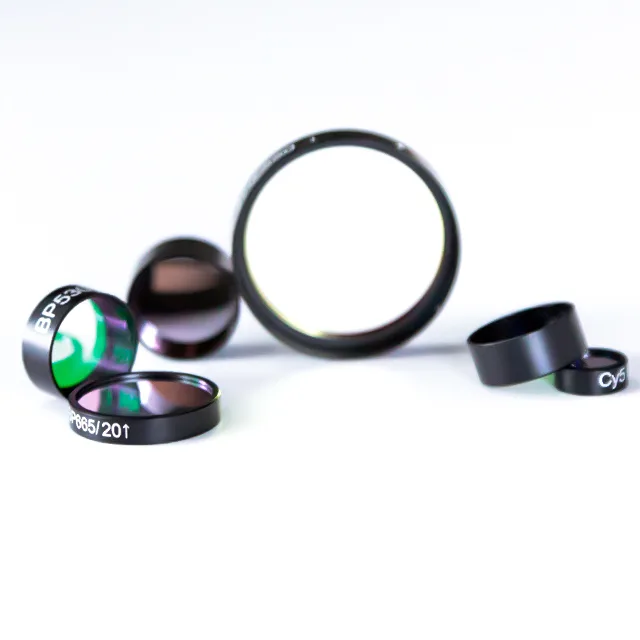
Bandpass filters allow light within a specific wavelength range to pass while blocking light outside that range. These filters are often used to improve contrast and reduce the effects of stray light in imaging applications.
By carefully selecting the passband range, bandpass filters can improve the signal-to-noise ratio, making them ideal for applications where clarity and contrast are critical. The challenge with bandpass filters is to precisely match the filter’s passband to the spectral characteristics of the light source and imaging target.
4. Longpass and Shortpass Filters
Longpass filters transmit wavelengths longer than the specified cutoff while blocking shorter wavelengths. Conversely, shortpass filters transmit shorter wavelengths and block longer wavelengths.
These filters are often used to separate different spectral regions or to block unwanted excitation light in fluorescence applications. Longpass and shortpass filters help improve image contrast by selectively allowing or blocking certain wavelengths, enhancing the visibility of specific features in the image.
How to choose the right filter
Selecting the right filter for biomedical imaging requires careful consideration of several factors, including the purpose of the imaging, the light source, and the spectral properties of the fluorophores or dyes used.
1. Imaging purpose
The choice of filter depends heavily on the specific imaging technique and its objectives.
Diagnostic imaging: In diagnostic imaging modalities such as computed tomography (CT) or magnetic resonance imaging (MRI), filters enhance contrast and improve visualization of specific anatomical structures. For these applications, it is best to use filters that maximize image clarity and minimize noise.
Research imaging: In research environments such as fluorescence microscopy or optical coherence tomography (OCT), the choice of filter depends on the need to selectively visualize specific molecules or structures. Narrowband or bandpass filters are often used to isolate specific fluorescent signals or to enhance contrast in complex samples.
2. Light sources and fluorophores
The type of light source used in the imaging system also affects the choice of filters.
Type of light source: Different light sources (e.g., LEDs, lasers, or halogen lamps) have unique spectral outputs. Filters must be matched to the spectral characteristics of the light source to ensure optimal performance. For example, laser-based systems typically require narrow-band filters that precisely match the wavelength of the laser emission.
Fluorophore excitation and emission: In fluorescence imaging, filter selection must take into account the excitation and emission spectra of the fluorophore. Correctly matching the excitation filter to the absorption spectrum of the fluorophore and the emission filter to the emission spectrum is critical to maximizing signal intensity and minimizing background noise.
3. Considering Signal Strength and Background Noise
The balance between signal strength and background noise is another key factor in filter selection.
Signal Enhancement: Filters enhance the desired signal while blocking unwanted light, thus improving overall image quality. This is especially important in applications with weak signals, where background noise can severely impact the visibility of target features.
Background Noise Suppression: Reducing background noise is critical to obtaining high-contrast images. Filters that effectively block out-of-band wavelengths or stray light sources can greatly enhance image clarity and contrast.
Key Performance Parameters of Filters
When selecting a filter, it is important to consider several key performance parameters that may affect its suitability for a particular application:
1. Optical Density (OD)
Optical density refers to the ability of a filter to block unwanted light. The higher the OD value, the greater the blocking ability, which is critical for minimizing background noise and stray light in high-contrast imaging applications.
2. Transmission
The transmittance of a filter indicates the percentage of light of a specific wavelength that passes through the filter. Filters with high transmittance at the desired wavelength provide better signal intensity, which is particularly important in low-light conditions or when capturing weak fluorescence signals.
3. Wavelength Range
The wavelength range of a filter determines which part of the light spectrum it transmits or blocks. Selecting a filter with the appropriate wavelength range is critical for optimizing image contrast and clarity.
4. Angular Sensitivity
Angular sensitivity refers to the performance of a filter relative to the angle of incidence of light. Filters with low angular sensitivity provide consistent performance over a range of angles, making them suitable for imaging systems with complex light paths or multiple light sources.
Filter Materials and Manufacturing Techniques
The materials and manufacturing processes of filters have a significant impact on their optical properties and durability:
1. Material Selection
Common materials used for filters include quartz, and plastic, each of which has its advantages and limitations.
Quartz: Quartz has excellent UV transmittance and high thermal stability. Its high optical clarity and durability make it ideal for applications where UV transmission is required or in high-temperature environments.
Plastic: Lightweight and low cost, but may have lower optical quality and durability than glass or quartz.
3. Manufacturing Techniques
Filters are manufactured using a variety of techniques, each of which affects their performance and suitability for different applications.
Dielectric coating: Involves depositing multiple layers of dielectric materials to achieve specific spectral characteristics. This method allows precise control of the transmission and blocking characteristics of the filter but is more expensive.
Dyed filters: Dyes are added to the filter material to absorb specific wavelengths. These filters are generally less expensive but may have lower transmittance and durability than dielectric-coated filters.
Best Practices for Using Filters
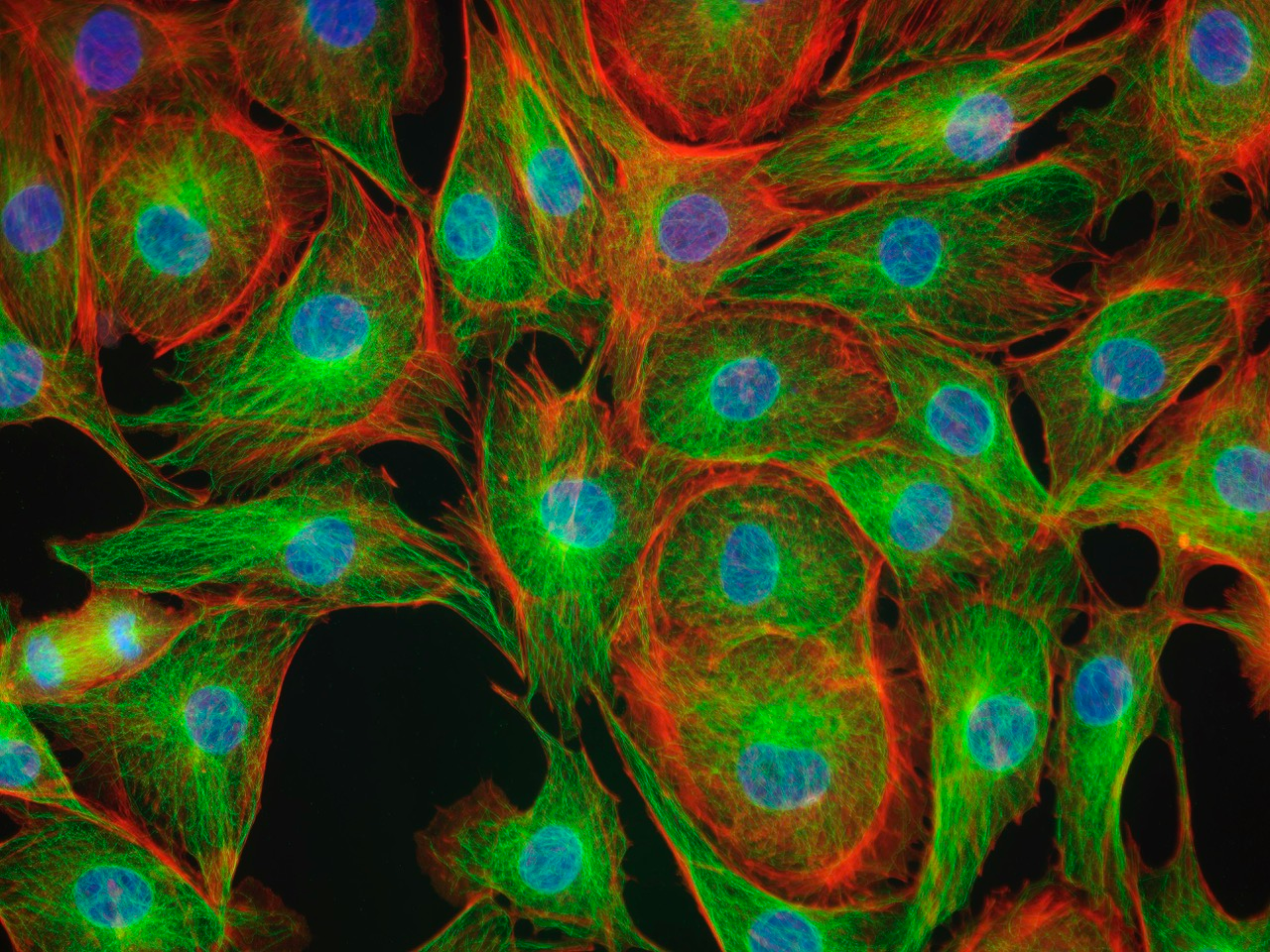
To maximize the life and performance of optical filters in biomedical imaging, it is important to follow best practices for their use and maintenance:
1. Maintenance and Cleaning
Proper maintenance and cleaning of filters can effectively prevent contamination and damage. Use a mild, non-abrasive cleaner and a soft cloth or lens tissue to clean filters, and avoid scratches or residue buildup, which can degrade performance.
2. Storage Conditions
Store filters in a clean, dry environment away from direct sunlight and extreme temperatures. Use a protective case or cover to prevent dust and moisture from accumulating on the filter surface.
3. Common Problems and Troubleshooting
Regularly inspect filters for signs of wear or damage, such as scratches, chips, or discoloration. Replace damaged filters promptly to avoid compromising image quality.
Troubleshoot performance issues by verifying that the correct filter is being used for the specific application and that it is properly installed in the optical path.
Conclusion
Choosing the right biomedical imaging filter can make the difference between obtaining high-quality images that meet specific application needs. By understanding the different types of filters, their applications, and the key factors that influence filter selection, researchers and medical professionals can make informed decisions to improve imaging performance and accuracy.
As biomedical imaging technology continues to advance, the demand for specialized filters will continue to grow, highlighting the importance of understanding the latest advances and best practices in filter selection and use.
Related reading: How Dichroic Mirrors Split Light