Fluorescence microscopy is a powerful technique used in biological imaging to observe and analyze cellular structures and processes. By using fluorescent dyes or markers that emit light when excited at specific wavelengths, researchers can observe cellular components with a high degree of specificity.
Fluorescence filters play a key role in this process as they help isolate the appropriate wavelengths for excitation and emission, ensuring that only the desired fluorescent signal is detected while minimizing background noise. This article will explore the different types of fluorescence filters and their impact on biological imaging.
Types of Fluorescence Filters
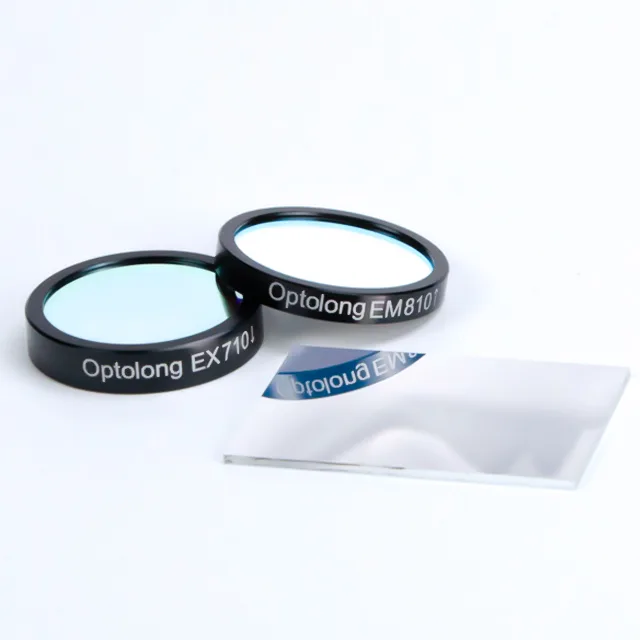
There are three main types of fluorescence filters, each with a different purpose in a fluorescence microscopy setup:
Excitation filters: These filters control the wavelength of light reaching the sample. They only allow a specific range of wavelengths to pass through and excite the fluorescent molecules. The choice of filter has a direct impact on matching the excitation peak of the fluorochrome being used, and a poor match can result in poor signal intensity.
Emission filters: When fluorescent molecules are excited, they emit light with a longer wavelength. Emission filters are placed in the light path to isolate the emission light from the excitation light. They only allow the emission light to pass through to the detector, ensuring that background noise is minimized and the fluorescence signal is optimized.
Dichroic mirrors: These are specialized filters that reflect the excitation light while allowing the emitted fluorescence light to pass through. They are placed at a 45-degree angle to the light path and act as a beam splitter, directing the excitation light to the sample and the emission light to the detector. This allows for simultaneous illumination and detection.
Fluorescence Filter Properties
The following key properties of fluorescence filters determine their performance in biological imaging:
Bandwidth: The bandwidth of a filter refers to the range of wavelengths it transmits. Filters with narrow bandwidths allow only a small fraction of wavelengths to pass, providing high specificity for detecting specific fluorophores. On the other hand, broadband filters have a wider range and can be used to detect multiple fluorophores, but may introduce more background noise.
Transmission efficiency: This property indicates how much light is transmitted through the filter. The higher the transmission efficiency, the more light reaches the detector, resulting in a brighter image. In fluorescence microscopy, high transmission efficiency allows for more accurate detection of weak signals, especially in low-light or live cell imaging conditions.
Blocking power: The ability of a filter to block unwanted wavelengths is called its blocking power. Effective blocking can reduce background noise and improve the contrast of the final image. Filters with high blocking power will minimize the leakage of excitation light into the detection channel.
Comparison Criteria
When comparing fluorescence filters, several factors should be considered to ensure optimal performance for biological imaging:
Spectral range compatibility: The spectral range of the filter must match the excitation and emission peaks of the fluorescent dye being used. For example, if the dye emits light at 520 nm, the peak transmission of the emission filter should be near that wavelength.
Filter quality and coatings: Advanced coatings on filters can significantly improve their performance, increasing durability, transmission efficiency, and blocking capabilities. Hard-coated filters tend to have longer lifespans and better optical performance than soft-coated filters.
Microscope compatibility: The filter should be compatible with the specific microscope setup, whether it uses filter cubes, filter wheels, or other mechanisms. This ensures that the filter will fit correctly and function optimally in the system.
Application of Fluorescence Filters in Bioimaging
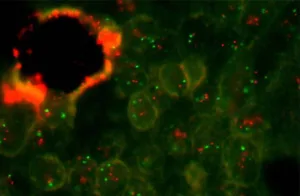
Fluorescence filters are used in bioimaging technology to optimize the performance of optical systems, improve image quality, and ensure the reliability of experimental data. The following are various applications of fluorescence filters in the field of bioimaging:
1. Live Cell Imaging
In live cell experiments, filters need to allow sufficient excitation and emission light to pass through to capture real-time cellular changes while minimizing photobleaching and phototoxicity to protect cell health. High-performance filters with narrow bandwidths can suppress background noise while detecting fluorescent signals, improving the resolution and contrast of dynamic cellular processes.
This is particularly important for studying real-time processes such as cell migration, signal transduction pathways, and protein interactions. For example, high-quality fluorescence filters can reduce photobleaching rates by 30% to 50%, significantly extending the duration of experiments.
2. Multicolor Imaging
When multiple fluorescent dyes are used simultaneously to label different molecules in the sample, the choice of fluorescence filters becomes particularly critical. Multicolor imaging requires filters with specific spectral characteristics to avoid spectral overlap (crosstalk) of different fluorescent dyes, to accurately distinguish fluorophores.
Filter sets typically include a combination of excitation filters, emission filters, and dichroic mirrors. By optimizing the spectral selectivity of these components, various targets in the same sample can be displayed. For example, in a five-color imaging experiment, an optimized filter set can increase the signal separation of different fluorophores to more than 90%, significantly reducing the effects of crosstalk.
3. Confocal and super-resolution microscopy
Advanced microscopy techniques such as confocal microscopy and super-resolution microscopy require filters with precise spectral characteristics to achieve higher imaging resolution and clarity.
The fluorescence filters in these systems must not only effectively transmit specific wavelengths of light, but also eliminate out-of-focus light (light that is not in the focal plane) and scattered light, improve the signal-to-noise ratio, and obtain more precise structural details.
For example, in super-resolution microscopy, customized narrow-band filters can achieve spectral resolution of sub-20 nanometers, which helps to reveal the subcellular resolution of ultra-cellular structures.
Choosing the Right Fluorescence Filter
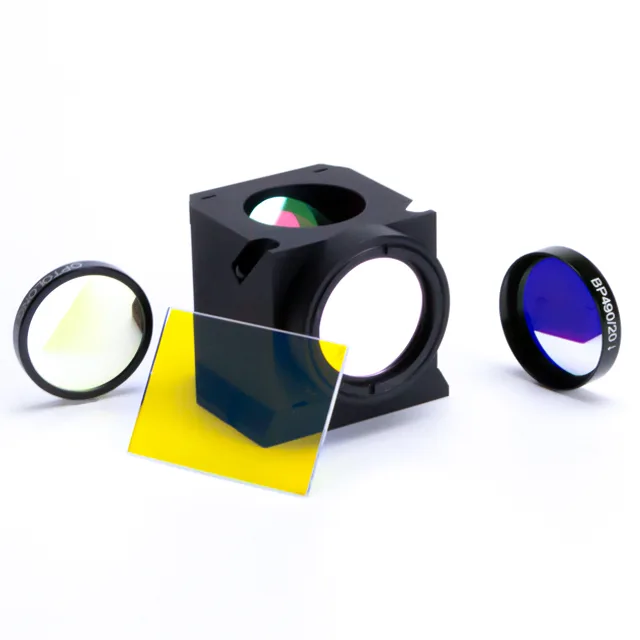
When choosing the right fluorescence filter, you need to focus on the experimental requirements and the characteristics of the fluorescent dye. You can refer to the following factors:
Choose based on dye characteristics: Different fluorophores have specific excitation and emission wavelengths. Matching the transmission range of the filter to these wavelengths ensures that the maximum amount of light is captured, thereby enhancing signal strength.
Imaging speed and sensitivity: Filters used in high-speed imaging devices (such as high-speed cameras or live cell monitoring) should have high transmission rates to capture rapid changes. For high-sensitivity applications (such as detecting low-abundance molecules), filters with minimal light loss and strong blocking capabilities are essential.
Custom filter options: Some manufacturers offer custom-designed filters for specific applications, such as unique spectral requirements or special imaging conditions. For example, Optolong provides customized fluorescence filters for a variety of biological and medical imaging needs, allowing researchers to optimize their optical systems for specific tasks.
Conclusion
Fluorescence filters are important tools for biological imaging that help researchers separate specific wavelengths to optimally detect fluorescent signals. Their properties, such as bandwidth, transmission efficiency, and blocking ability, have a significant impact on the quality of fluorescence microscopy images.
When selecting filters, factors such as spectral compatibility, coating quality, and system compatibility should be considered. As filter technology advances, fluorescence imaging will continue to evolve, providing more precise tools for exploring the complexity of biological systems.
In the future, improvements in filter materials and manufacturing techniques are expected to further improve filter performance, leading to better image quality and new applications in biological research.
Related reading: What are the parts of a microscope?